Medical imaging companies in Europe must comply with an array of national legislative standards, rather than a blanket regulatory framework, because the EU’s Medical Device Directive lacks specific technical standards. This has hindered innovation and made life difficult for medical imaging manufacturers there.
Three dimensional (3D) imaging – where data is processed in a computer and displayed on a 2D monitor to give the illusion of 3D – has evolved steadily in the last two decades. It is now being applied in a diverse range of medical fields: surgery, radiology, CT imaging, orthodontics, and oral and maxillofacial surgery.
In orthodontics, applications for 3D imaging include the pre- and post-orthodontic assessment of dental and skeletal relationships and facial aesthetics, the auditing of orthodontic outcomes with regard to soft and hard tissues, 3D treatment planning, and 3D soft and hard tissue simulation. 3D modelling and imaging can also be used in the fabrication of ‘archwires’, and the archiving of 3D facial, skeletal and dental records for treatment planning and research.
EVOLVING DISCIPLINE
The first commercial CT scanner appeared in 1972. It soon became apparent that a stack of CT sectional images could be used to generate 3D information. Researchers began investigating the use of 3D imaging in examining craniofacial deformities, and in1986 the first simulation was developed for craniofacial surgery.
3D imaging soon evolved into a discipline of its own, primarily focusing on various forms of visualisation, manipulation and analysis of multidimensional medical structures.
How well do you really know your competitors?
Access the most comprehensive Company Profiles on the market, powered by GlobalData. Save hours of research. Gain competitive edge.
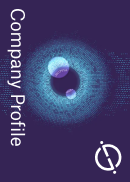
Thank you!
Your download email will arrive shortly
Not ready to buy yet? Download a free sample
We are confident about the unique quality of our Company Profiles. However, we want you to make the most beneficial decision for your business, so we offer a free sample that you can download by submitting the below form
By GlobalDataToday radiologists have to deal with an increasing volume of image processing, making a shift from image interpretation to management inevitable. Film is no longer used today, while visualisation and analysis techniques using volumetric tools have evolved from research tools into necessities.
POWERFUL TOOL
3D visualisation techniques now provide radiologists with the tools they need to carry out multi-planar reformation, maximum-intensity projections, shaded surface displays and volume rendering. However, users of 3D systems face challenges and limitations, such as a lack of trained personnel, and the time and expense involved in installing and operating a solution.
When 3D imaging was first introduced nearly two decades ago, it was only used as a research tool. Radiologists then started to familiarise themselves with the technology to help them examine body cross-sections and make correct diagnoses. Meanwhile, computers became faster and more user-friendly, and radiologists no longer felt intimidated by the technology. Today radiologists feel very much a part of a learning process that is rapidly changing the face of imaging and diagnosis.
The main restraint or challenge faced by radiologists is the time needed to learn about the new technology and understand the different 3D applications. Another problem is finding the staff required to manage the new technology. Even when technologists are available, they often need to be trained in the latest 3D image processing techniques. The technology takes several months to learn, usually on the job, and technologists need to have plenty of practice.
For the subjective assessment of deformities, 3D models are valuable for locating the source of a deformity and its magnitude. They can be manipulated in any direction, providing the orthodontist or surgeon with considerable information without recalling a patient or extending the clinical assessment time required. An outcome assessment can be performed by visually comparing pre- and post-treatment models placed side by side.
3D IMAGING TECHNIQUES
In 2D photographs or radiographs, there are two axes (the vertical x-axis and the horizontal y-axis axis). The Cartesian coordinate system in 3D images consists of the x-axis (the transverse dimension), the y-axis (the vertical dimension) and the z-axis (the antero-posterior dimension or ‘depth axis’). These three axes together define a space or volume in which multidimensional data can be represented. This is called the 3D space.
3D models are generated in the following steps:
- Modelling. Mathematics is used to describe the physical properties of an object. The modelled object is usually seen as a wireframe consisting of triangles or polygons. The modelling procedure is completed with the addition of a surface (a layer of pixels on the object), a procedure called image mapping or texture mapping.
- Shading and lighting. Shading and lighting is added, bringing realism to the 3D object.
- Rendering. The final procedure is called rendering, where the computer converts the anatomical data collected from the patient into a realistic 3D object to be viewed on a computer screen.
3D imaging approaches can be classified into three categories:
- Slice imaging. This process uses a set of CT axial data to reconstruct 2D images.
- Projective imaging. This is a laser scanning technique that produces a ‘2.5D’ image.
- Volume imaging. This category includes holography or varifocal mirror techniques.
Different measurement techniques can be employed in 3D imaging:
- 3D cephalometry. 3D cephalometric is a research-oriented procedure. The technique is time-consuming, it exposes the patient to radiation and it cannot define soft tissues. It can be difficult to relate the technique to the landmarks obtained from a radiograph.
- 3D CT scanning. This technique has gained popularity in medical applications. Its main disadvantages are that patients are exposed to high doses of radiation, only limited resolution of facial soft tissues is possible and artifacts can occur, due to the presence metal.
- 3D laser scanning. Laser scanning provides a less invasive method of capturing facial texture and landmarks. However, the technique has several shortcomings as a facial scanning technique: the process is time-consuming, which can result in distortions in the scanned image; there are safety issues related to the exposure of patients’ eyes to a laser beam; and the technique cannot capture soft tissue surface texture, making the identification of landmarks that are dependent on surface colour difficult.
- Moiré topography. Moiré topography delivers 3D information based on facial contour fringes and fringe intervals. However, the best results can only be obtained on faces with smooth contours, while care needs to be taken in positioning the head, as any small change in position can produce pronounced changes in the fringe pattern.
- Structured light techniques. This is a light-based technique where the object is illuminated by a light pattern and only one image is required. The position of illuminated points in the captured image compared with their position on the light projection plane provides the information needed to extract features on the imaged object. To get high-density models, the face needs to be illuminated several times with random light patterns. This increases the capture time and the risk of head movements. Also the single camera cannot provide a 180° (ear-to-ear) facial model. This requires either the use of several cameras or the rotation of the subject around an axis. This procedure, being a very elaborate one, is not always practical.
- Vision-based scanning techniques. These techniques use non-invasive, non-contact vision-based imaging systems.
- Stereophotogrammetry. Two cameras are deployed in stereophotogrammetry to recover 3D information on surface features using triangulation. The technique has been applied clinically using a portable stereometric camera optically linked to a plotting instrument. The latest computer technology has allowed stereophotogrammetry to process complex algorithms in order to convert simple photographs into 3D measurements of facial changes.
- 3D facial morphometry. Although this is not a true imaging system, it uses two CCD cameras to capture the subject, while real-time hardware is used to recognise markers. Software, for the 3D reconstruction of landmarks’ x, y, and z, coordinates the markers relative to the reference system. Landmarks are located on the patient’s face and then covered with 2mm hemispheric reflective markers. An infrared stroboscope is used to illuminate the reflective markers. Acquisitions are required from both sides to capture the whole face.
Unfortunately, the placement of landmarks on the face is time-consuming and labour-intensive, while a change in facial expression can cause errors. As a result, this system cannot be used as a 3D treatment planning tool or as a communication media with orthodontic or orthognathic patients.
EUROPEAN REGULATIONS
Radiology is subjected to stringent regulation on both the supply and the demand side in Europe. The legislation includes safety directives for manufacturers, hospitals and physicians, covering areas such as electromagnetic compliance, data protection and radiation exposure.
All market participants must fulfil their regulatory obligations and provide equipment that enables end-users to fulfil their requirements. 3D imaging that requires radiological scanning must comply with rules designed to ensure patient and radiology staff safety. Patient and staff safety must also be safeguarded by ensuring that staff are adequately trained.
Certain EU members severely restrict the levels of ionizing radiation exposure they allow. However, the levels of radiation used for medical imaging are partly determined by the economic situation in each member state, the equipment available, and the prevailing culture and practice in the radiation protection field in individual states. Concern over radiation protection is high in Germany and Scandinavia, moderate in the UK, France and Spain, and low in Italy.
Regulation has disadvantages: it increases the cost of a product, prolongs time-to-market and requires additional administrative procedures. Sometimes regulation limits flexibility, and it often complicates product line management. However, the lack of a consistent regulatory framework across the EU has left medical imaging vendors struggling to tailor their equipment and solutions to the requirements of individual regions and hospitals.
There is a strong feeling among vendors that the complete integration of regulatory standards across Europe will be of tremendous benefit to the medical imaging industry. Regulatory harmony would also help remove barriers to trade and establish a level playing-field for all European nations. This could result in greater collaboration between countries in terms of technical, regulatory and infrastructural development.