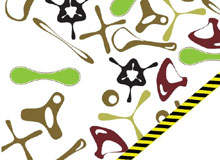
Medical devices such as stents and catheters play a substantial role in the treatment and management of urinary incontinence in community and hospital settings, with approximately 200 million catheters used throughout the US each year, as shown in Table 1. Clinically, urinary catheters are used either intermittently or may be left in position for prolonged periods (indwelling catheterisation), sometimes as long as three months. During these long indwelling periods maintaining the device integrity and hence the clinical performance is extremely difficult.
Medical device research is developing innovative materials to improve the inherent complications associated with indwelling systems. Meeting this challenge is of particular importance for urinary devices given that 90% of all patients undergoing long-term catheterisation develop bacteriuria within four weeks. Of these 90% of patients, 10–25% develop a urinary tract infection (UTI), accounting for 40% of all hospital-acquired infections, with a typical cost to the UK’s NHS of approximately £1bn/year, a figure that could be significantly reduced should suitable materials become available.
Medical device-associated infections
The problems associated with implanted urinary devices and the significant role microbial biofilms play in infection are now widely recognised as major disadvantages of an otherwise highly effective treatment strategy. Although there are many factors influencing the lifetime of urinary devices, the most notable cause of failure is commonly related to microbial biofilm formation, encrustation and recurrent urinary tract infection.
The development of the microbial biofilm, defined as microbially derived sessile community of microorganisms irreversibly attached to a surface and housed within a protective matrix of exopolymers that they have produced, is a significant drawback.
Immediately following insertion the device surface becomes primed for infection through ‘surface conditioning’. During this process, adsorption of host-derived proteins, extracellular matrix proteins and coagulation products mask the underlying material surface. This results in a material that is more susceptible to bacterial absorption.
How well do you really know your competitors?
Access the most comprehensive Company Profiles on the market, powered by GlobalData. Save hours of research. Gain competitive edge.
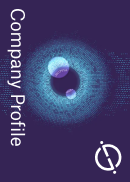
Thank you!
Your download email will arrive shortly
Not ready to buy yet? Download a free sample
We are confident about the unique quality of our Company Profiles. However, we want you to make the most beneficial decision for your business, so we offer a free sample that you can download by submitting the below form
By GlobalDataThe reversible attachment of the microorganisms, followed by the secretion of extracellular polymeric substances (EPS), results in cell-to-cell bridges, cementing the cells to the surface. Once colonisation has been established, the bacteria will grow and divide, resulting in the formation of microcolonies. Other freely suspended cells will become entrapped and the microbial biofilm develops.
Biofilm growth and biofouling are global problems that have a tremendous economic impact in medicine and industry. The cell-to-cell communication occurring in biofilm-associated bacteria has been shown to regulate virulence in numerous human and plant pathogens and is crucial for maintaining a pathogenic or symbiotic interaction of a variety of microorganisms with their hosts.
The CDC and FDA state more than 80% of human infections involve biofilms. Globally, biofilm-derived infections affect more than 25% of all hospitalised patients, with a 5–10% daily incidence of urinary tract infections in patients with urinary catheters and virtually all patients who undergo long-term catheterisation (≥28 days) becoming infected.
The susceptibility of implantable medical devices to biofilm formation is a major obstacle. Biofilms are characterised by their inherent tolerance to biocides, antimicrobials and clearance by humoral or cellular host defence mechanisms as antigens are hidden and key ligands are suppressed. It can require as much as thousand-fold more antibiotic/biocide to eradicate a biofilm than a planktonic population.
The major mechanisms of biofilm resistance are a slow penetration of biofilm exopolymers by antibiotics, a heterogenous growth rate within the biofilm, an increased rate of genetic transfer, expression of resistance genes, hypermutation and the multicellular nature of the biofilm itself.
Therefore, in addition to providing protection from mechanical removal of microorganisms from the infection site, the biofilm also confers tolerance to the microorganisms within. Microorganisms are maintained in a localised, privileged microenvironment with concentrated enzymes and metabolic products, resulting in a microbial population that is less metabolically active than equivalent planktonic (free-floating) bacteria, and so is largely unaffected by antibacterial agents, whose mechanism of action frequently depends on the metabolic activity of the bacteria.
The catheter surface lends itself to bacterial adherence and migration in two ways – the extraluminal route and the intraluminal route. The extraluminal route begins when the bacteria are directly introduced to the extraluminal surface during and after insertion. These tend to be endogenous microorganisms from the gastrointestinal tract, migrating from the perineum to the urethra after the catheter has been inserted. Passage through the intraluminal route occurs when bacteria gain entry through a failure in the closed drainage system or through contamination of the drainage bag.
Biofilm formation and encrustation
Encrustation of devices is a primary complication, with overall incidence as high as 58%. It can occur either in the presence or absence (metabolic encrustation) of infectious organisms. However, it is primarily associated with infection by urease-producing bacteria such as Proteus mirabilis, biofilm formation and subsequent biomineralisation.
Biomaterial surface colonisation with urease-producing bacteria causes alkalinisation of the urine and the biofilm matrix, and lowers the solubility of struvite and hydroxyapatite in the urine and the immediate milieu of the biofilm. The urease mediated hydrolysis of urea liberates ammonia and carbon dioxide:
Hydroxide formation from the reaction of ammonia and water, despite the presence of weakly acidic carbonic acid, results in an overall elevation of urine pH and the subsequent precipitation of struvite and hydroxyapatite from urine which is deposited on the biomaterial surface.
Encrustations associated with an infectious origin are therefore primarily composed of magnesium ammonium phosphate (struvite) [MgNH4PO46H2O] or calcium phosphate (hydroxyapatite) [Ca10(PO4)6(OH)2], as shown in Figure 1.
Encrustation is a common occurrence with long-term catheterisation and can have serious consequences for patient care, potentially resulting in complete occlusion of the catheter lumen. One study showed that in patients with long-term indwelling catheters, 48% developed a problem with catheter blockage and 37% experienced bypassing, whereby the urine passes along the outside of the catheter, a potentially painful experience. These blockages can limit the time in which a device can remain in situ, causing more frequent changing of the device, and cause trauma to the urethra and bladder on removal. Other serious consequences include septicaemia, pyelonephritis and shock.
Controlling infection
Aseptic technique and adherence to hygienic principles by trained healthcare professionals are the first steps in reducing infection in patients hosting urinary medical devices. The only interventions that have been found to be consistently successful are reducing the duration of the indwelling catheter, strict maintenance of a closed drainage system and prophylactic antibiotic therapy. However, the latter carries the risk of microbial resistance, a recent concern.
Silicone and latex are the two most commonly used materials for manufacturing catheters. While both of these materials offer desirable mechanical properties, neither offers significant resistance to bacterial adherence or encrustation. It has also been previously reported that latex only offers a usable lifespan of 14 days. Catheter coatings have been developed for improved biocompatibility and decreased adherence and encrustation.
Although several coatings have been investigated, the most commonly reported are hydrogels such as poly(2-hydroxyethyl methacrylate), polyvinylpyrrolidone and polyethylene oxide. They offer reduced coefficient of friction, improved patient comfort and lower cell adhesion, as well as greater patient acceptability, lower levels of urinary leakage and longer lifespans.
Efforts to develop novel urinary devices are now focused on strategies that resist bacterial colonisation through the use of bioactive (biocides or antibiotics) biomaterials. The simplest approach involves immersion of the catheter in an antimicrobial solution before insertion, which has been shown to significantly reduce bacterial adhesion. A drawback of this method is that the local drug concentration will not remain high over long periods. However, short-term resistance may be achieved, giving some protection against infection via the extraluminal route.
After implantation, therapeutic agents may be released from the biomaterial and ultimately reside locally in the biological fluid adjacent to the device, preventing bacterial adherence. Silver has been investigated for use as a bioactive biomaterial coating due to its activity against Gram-positive and Gram-negative bacteria. Its use has been the subject of debate following conflicting studies. Interestingly the FDA has approved two hydrogel silver catheters – Bardex® IC and LubriSil IC, latex and silicone-based catheters respectively. Both consist of a lubricious hydrogel coating over a layer of metallic silver on the inner and outer surfaces of the catheter.
Other bioactive approaches include the use of antimicrobials, antibiotics and combination therapies. Triclosan, a well-accepted chlorophenol compound often used in many other healthcare products (handwashes, toothpastes) has been shown to inhibit biofilm growth of several key pathogens. Moreover, a hydrogel coating containing liposomes of ciprofloxacin significantly reduced bacterial adhesion of Pseudomonas aeruginosa over a seven-day period.
Norfloxacin has also demonstrated significant inhibition of E.coli for 30 days whereas gentamicin sulfate reduced biofilm formation and bacteriuria in animal models. Heparin has shown promise as an effective crystallisation inhibitor, slowing encrustation compared to uncoated devices.
Urology for the future
Despite the attempts to alleviate the complications that plague the use of urinary devices, many problems still exist. While antibiotic therapies and novel surface coatings may provide a temporary resolution, the only definitive solution is device removal.
More intelligent biomaterials and devices would offer benefits of superior performance and improved dwell time, to be more patient acceptable and significantly curb increasing healthcare costs.
Research at The School of Pharmacy, Queen’s University Belfast is focused on addressing the serious life-threatening infections arising from the implantation of medical devices and in particular urinary catheters.
In the industrialised world scientific and medical advances have improved patient care and extended population life expectancy and quality of life. However, in an ageing population the proportion of adults requiring therapeutic interventions involving the use of indwelling medical devices continues to rise. The ubiquitous nature of medical device-associated infections continues to burden healthcare providers with increased patient morbidity and mortality.
Urinary medical device design has come a long way since the first described use of papyrus catheters in ancient Egypt. Inarguably, urological devices are effective and economical solutions to a wide range of urinary tract conditions and their use has become an important cornerstone of clinical urology.
Despite this, the use of such devices is associated with infection, biofilm formation and encrustation. Since the invention of the Foley catheter in 1936, incremental improvements have been made in addressing the problems associated with their use. However, no definitive breakthrough has yet been made which allows long-term, infection-free employment of these devices in the clinical setting. Research groups from around the world are developing new and innovative ways to combat infection and reduce the patient morbidity and mortality, risk and associated costs of infection.
Research material has been referenced in this text. For full details please contact the editor, andrewtunnicliffe@spgmedia.com