Towards the end of the last century, spinal and orthopaedic implants evolved towards progressively stronger and stiffer devices, as it was presumed that increased construct rigidity would optimise the biological milieu and provide more rapid and robust healing and arthrodesis.
Within the last decade, however, our understanding of the biology of bone healing and fixation has resulted in a re-evaluation of this potentially flawed tenet.
Based on Woolf’s law of bone healing, it has become evident that dynamic fixation implants that provide more optimal load-sharing profiles can actually result in earlier and more effective fusion rates than traditionally stiffer constructs. This concept evolution is reflected in the spinal market, where dynamised or load-sharing anterior cervical plate constructs and semi-rigid posterior pedicle-rod fixation systems have become increasingly popular among clinicians.
DEGREES OF STIFFNESS
Numerous recently published studies have demonstrated that fusion rates with load-sharing implants are generally equivalent to, if not superior to, those seen with traditional rigid stabilisation systems.
Although the very nature of orthopaedic and spinal implants requires an inherent degree of stiffness and stability to treat what is typically a mobile, deformed or unstable pathology, it would seem that this aim must be weighed against the negative consequences of excessive stress-shielding, which can lead to a sub-optimal biological environment for healing. An optimal implant would thus be strong yet flexible with a biological modulus similar to that of the native bone to which it is fixed.
How well do you really know your competitors?
Access the most comprehensive Company Profiles on the market, powered by GlobalData. Save hours of research. Gain competitive edge.
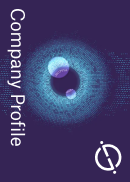
Thank you!
Your download email will arrive shortly
Not ready to buy yet? Download a free sample
We are confident about the unique quality of our Company Profiles. However, we want you to make the most beneficial decision for your business, so we offer a free sample that you can download by submitting the below form
By GlobalDataComposite flow moulding (CFM) technologies, available from companies such as Icotec, are exciting examples of the use of novel polymeric materials to resolve the problem of traditional metallic implants, which are inherently much stiffer than is needed to achieve surgical fixation and correction of the far more pliable and softer bone in the patient.
In addition to the issue of the overly rigid stiffness of metallic stabilisation constructs, recent attention has been focused on the metallic fixation members or the bone screws themselves. Classically, screw pullout, dislodgement and breakage have been attributed to a failure either of the mechanical strength of the overall construct or the biological potential for bone healing and arthrodesis.
Whereas construct failure was more common in early-generation implants, present day fixation systems are typically more robust and rigid enough to reduce and immobilise the target spinal or orthopaedic pathology, provided that the primary bone fixation does not fail.
IMPLANT FAILURE
As the systems themselves are now so well engineered, the vast majority of implant failures now occur at the bone-screw interface with screw pullout being the most common pathomechanistic cause of construct failure. For this reason, industry and clinicians alike have become focused on optimising the integrity of the bone-screw purchase through a variety of surgical and manufacturing techniques.
When the bone of the treated segment is strong and healthy, the initial fixation of traditional spinal and orthopaedic screws is excellent, with more than adequate pullout strength. However, micromotions resulting from the normal range of motion within the human skeletal system inevitably lead to a progressive degradation from the initially implanted state.
With successful healing of the bone across the mobile segment, these micromotions are dissipated, thereby leading to long-term stability of the bone-screw interface and, by extension, the overall construct and segment integrity.
However, should the bone fail to heal, these micromotions will persist and cause the metallic screw to oscillate within the far softer surrounding bone interface. Indeed, when subjected to persistent toggling the 10-100x modulus mismatch of the metal to cortico-cancellous bone interface will predictably result in destruction of the bone against the metal, radiographic lucency around the screws and loss of pullout resistance overall.
IMPLANT-BONE INTERFACE
Surface treatment techniques such as hydroxyapatite-coating or rough blast-finishing of the metallic interface have been used extensively in orthopaedics to provide better bone-metal interface bonding and cohesion. Polymethylmethacrylate (PMMA) cement augmentation has also been used widely to augment the initial fixation strength of screws within bone, particularly in situations such as osteoporosis.
However, long-term studies have demonstrated that the PMMA-bone interface will ultimately degrade just like the metallic bone-screw interface if fusion does not occur.
Such techniques have been used with great success to maintain the position of large total joint and spinal segment arthroplasty-type devices. In the long bone and spinal implant field, however, these techniques have met with less success for several reasons, including the smaller size and surface area of these screws, the cancellous bone that the screws often reside in and the often orthagonally oriented pullout forces that are vectored through the long lever arm of the screw.
Overall, it has become evident that simple surface-treatment and cement augmentation techniques, in and of themselves, will not solve the issue of screw loosening over time, should fusion fail and motion persist.
The critical nature of this concept has been recently magnified in the spinal industry, where motion preservation has come to be increasingly utilised to treat degenerative disorders.
Numerous dynamic fixation systems have been introduced to stabilise the injured or diseased painful motion segment while still allowing for some degree of natural motion. The concept of restoring this ‘neutral zone’ of stability and motion has been supported by numerous clinical and biomechanical publications.
The majority of these posterior dynamic stabilisation systems still rely on traditional pedicle bone screws as their primary means of fixation to the spinal vertebrae. Accordingly, these cutting-edge implants are subject to identical loads and stresses with the same resultant mechanisms of failure observed in traditional pedicle screw-based fusion systems.
As dynamic spinal arthroplasty procedures are typically done without concomitant arthrodesis or fusion, it becomes evident that failure at the pedicle screw-bone interface will prove to be even more of a problem in arthroplasty constructs, as there will never be immobility from successful fusion.
The issue of failure at the bone-screw interface thus remains the fundamental underlying problem of the existing spinal and long-bone fixation methods utilised in both rigid fusion and arthroplasty-type procedures.
NEW IMPLANTS REQUIRED
To minimise these issues of the screw-bone interface, a fundamental revision and redesign of the pedicle or bone screw itself is required.
As the modulus mismatch of the metal to the much softer bone is the Achilles heel of both fusion and arthroplasty systems, it is clear that the hardness of the screw within the bony environment must be reduced without excessive sacrifice of its strength, rigidity and early fixation strength.
Examining the variety of pure and alloy metals presently used in orthopaedic and spinal implants, it is unlikely that any metallic-based screw will be able to approach the ideal modulus of the cortico-cancellous bone environment. For a more optimal solution, novel material-type polymers will be needed to optimise the material properties of today’s bone screws.
The screws and implants manufactured via the CFM technology process are a good example of just such a solution to the critical issue of the bone-screw interface modulus mismatch.
APPLICATIONS OPTIMISED BY CFM
Based primarily on pultruded rods with endless carbon fibre and a PEEK matrix (ECF-PEEK), CFM technology utilises a controlled thermoplastic process to pultrude endless carbon or glass fibres through the base material to create implants with many features that make them optimal for spinal and orthopaedic implant formulations.
PEEK material has a well-established history in spinal implants, with attractive features including low weight, biological inertness, radiolucency, high compressive strength, corrosion resistance and excellent fatigue resistance.
Accordingly, it has become the synthetic material of choice for load-bearing constructs such as vertebral body spacers and inter-body cages.
By itself, however, PEEK’s tensile properties are not ideal for use in plates and screws, as it is unacceptably brittle and cannot meet the stress, shear and torque requirements of such implants. By adding as many carbon fibres as possible during the pultrusion process, this material limitation of pure PEEK is overcome as these ‘endless’ strands provide a strong, flexible, internal endoskeleton to the resulting ECF composite.
Like reinforced concrete, the ECF composite material is extremely strong and flexible, yet has a modulus that is far more ideally suited for use in bone as compared to traditional titanium and steel screws.
With this improved modulus match, it is logical to assume that ECF-based screws will be able to more effectively dampen forces seeking to dislodge it from within the bony matrix, thereby leading to more stable fixation over a much longer period.
Finally, the CFM process allows for unique design opportunities allowing for controlled differential moduli and stiffness within a single implant. The endless fibres remain intact during the production process, resulting in an optimal fibre content in and around the screw holes of the long bone ‘Snakeplate’, thereby reinforcing these high-stress zones of the implant.
For these and a variety of other reasons, ECF composite may represent an optimal material by which to reinvent and redesign classical metallic screws and plates for today’s applications.
DEFINITIONS OF TERMS
- Pultrusion. Process of rod production (raw material) in which carbon fibres are pulled through a bath with matrix
- Composite flow moulding (CFM). Shaping of pultruded rods in a thermoplastic process into the final implant form
- ECF-PEEK implants (Endless carbon fibre with PEEK). A new generation of spinal implants formed in the CFM process