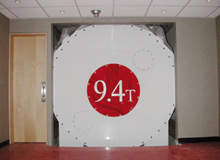
When the University of Illinois at Chicago (UIC) needed someone to be the first person to have their brain imaged with the 9.4 Tesla (T) magnetic resonance imaging machine, there was really only one candidate: Keith Thulborn, director of UIC’s Center for Magnetic Resonance Research and the man behind the project, stepped forward.
“Actually, it was anti-climactic,” says Thulborn. “And that’s a good thing. It’s the same as on the 3T scanner – everybody said I would be throwing up, would see flashing lights and I’d have a metallic taste in my mouth. None of that happened. The thing about each of these experiences is that it is a relatively benign experience and that was a really nice surprise.”
Tests carried out on members of the public proved equally positive: 25 healthy volunteers – 12 men and 13 women – were exposed to the 9.4T scanner and to a mock scanner with no magnetic field. Vital signs and cognitive ability were measured before and after both tests. There were no significant changes in heart rate, blood pressure, respiratory rate or other vital signs, nor were there any notable differences in the cognitive testing of volunteers following mock scanning (as opposed to real scanning). The researchers concluded that exposure to a 9.4T static magnetic field does not present a safety concern.
Tissue viability
The 9.4T machine has a number of intriguing possibilities, particularly concerning tissue viability. “It is very hard for physicians to tell whether a piece of tissue is viable,” says Thulborn. “They can tell if a patient is alive or dead. But if you want to know the health of a particular area of tissue – which might be in the heart, the brain or another organ in the body – being able to tell whether it is alive or dead is rather difficult until it becomes necrotic, and at that stage you’ve got a catastrophe on your hands.”
To intervene early in a disease process there has to be some measure of tissue viability. If a patient can’t maintain a sodium ion gradient, then the tissue is not viable. The higher magnetic field created by the machine offers greatly increased sensitivity to detect signals from non-proton elements such as sodium, carbon, oxygen, phosphorus and nitrogen in a reasonable amount of time for human imaging (less than ten minutes).

US Tariffs are shifting - will you react or anticipate?
Don’t let policy changes catch you off guard. Stay proactive with real-time data and expert analysis.
By GlobalDataThese signals offer a new biochemical dimension to MR imaging that allows a new set of metabolic questions to be addressed that may be a more direct way of making clinical decisions and monitoring disease.
Brain tumour prognosis
The 9.4T machine allows for a far greater degree of exactitude in treating illness. This new dimension of human metabolic imaging examines the cellular machinery directly at the site of a disease.
“An example of where we are applying this is in the treatment of brain tumours,” explains Thulborn. “The prognosis for the treatment of high-grade brain tumours has not changed in the last 30 years. We’ve done great things with many forms of cancer, but you’ve still got an 80% mortality rate in two years with a brain tumour. Our treatments today are totally inadequate. This is because if you survive two years, your chances of surviving 20 years are extremely good. It means that our first line of treatment doesn’t work and why don’t they work? Well, because we don’t know what we are doing.”
Surgery is used to de-bulk the tumour, radiation therapy to irradiate the surgical bed and then chemotherapy is applied. “But we don’t know whether we are succeeding for a particular tumour or not and we don’t localise our treatment in a way that would make sense based on the viability of the tumour,” says Thulborn. “We deliver the radiation uniformly over the surgical bed. If we knew one part of the tumour was responding and another part wasn’t responding, we would change the way we do the therapy.”
Being able to adapt radiation therapy based on tissue response during the therapy would presumably lead to more successful treatment, an area that sodium imaging allows. Response in the tumour bed can be seen in the first couple of weeks of treatment: treatment takes six weeks.
“So, we can tell which areas are responding and which are not,” says Thulborn. “You can use the information to change the way you are distributing it and hopefully get a better result.”
Early imaging
The results proved deeply satisfactory for Thulborn, who has been working on this project since 2000, although the roots of this particular machine go further back than that. “The goal 30 years ago was “what techniques do we have available that will allow us to examine biochemistry in the intact tissue?”” he says. “There aren’t that many ways you can do that. First of all, it has to be an imaging procedure; you don’t want to cut the organism open.”
Back then, positron emission tomography (PET) was used. An isotope was generated, which was produced in a very low concentration that wouldn’t perturb the cellular machinery, and that allowed images to be generated, based on a biochemical perimeter – glucose metabolism or oxygen consumption rate. This is a measure of the gross national product of the cellular machinery.
“The problem with PET is that there are only a limited number of perimeters,” explains Thulborn. “We always use radiopharmaceuticals and so we are always dealing with radioactivity, which makes it very hard to do human experiments. We’d like to study the development of a human as well as the degeneration.
“We’re dealing with brain function to look at how the brain develops, and how the normal adult brain works. Then we want to look at how brain function deteriorates with age.”
The development of nuclear magnetic resonance (NMR) in the late 1970s proved a vital step forward. “The only signal you could see at that stage looking at proteins was based on water,” says Thulborn. “Because biological systems are 80% water, we have managed to produce nice images in a reasonable amount of time that tells us about anatomy. We still had the problem that water is not the whole story.”
The environment in which the cellular machinery works is made up of the vital signs that come from other elements: carbon is the substrate that is oxidised to produce energy; cells maintain ion gradients with sodium and potassium; and many of the neurotransmitters involve the use of nitrogen. The other elements all have MR signals, but have very low sensitivity. So the quickest way to increase the sensitivity of an MR experiment is to increase the field strength with which the experiment is performed.
Field strength power
In the 1980s and 1990s, scientists sought to build increasingly powerful machines. Paul Bottomley, an engineer at General Electric (GE) at the time, wanted to build a 2T system. Although the machine only reached 1.5T, it showed that higher field strength was possible.
“At that time, there were a lot of very influential engineers who said it was impossible to do,” says Thulborn. “Bottomley’s machine was state-of-the-art for many years. When we got to the 1990s, there was an attempt in the US to change healthcare and make it cheaper. Imaging was one of the things that was escalating astronomically. The manufacturers said: ‘We have to go back to low fields. We’ll build cheaper magnets’. But when you build low field, the quality of the images declines.”
At this stage, Thulborn was seeking to do biochemical research and decided to build a 3T scanner. The University of Pittsburgh Medical Centre was prepared to fund him and the only company that could be persuaded to do it the way he wanted was GE. GE built the first 3T prototype scanner on its clinical platform and that gave access to sodium imaging: in this way quality sodium imaging could be done in less than ten minutes.
“Unlike the animal experiments where you can image for hours on a single animal, humans are never that cooperative,” notes Thulborn. “To have a clinically feasible MR study you have to be able to do it in less than ten minutes, hopefully much less: you’d like to do it in a couple of minutes. 3T led the way in demonstrating that sodium imaging was feasible, but sodium imaging is only the first step.”
Thulborn was driven by the desire to achieve higher sensitivity in order to detect signals from non-proton elements such as sodium. “In the brain, sodium is present at 40 millimole – remember you are dropping from 80 molar, which is water,” he says. “That drop in sensitivity is about 700-fold, so 3T really helps in providing reasonable resolution in a reasonable amount of imaging time. To do that we had to change the way we did the imaging.
“When we first started 3T, everyone said it would fail and patients would be suffering from side effects. Just as Paul Bottomley got a lot of criticism for building a 1.5T scanner, with 3T it was a case of ‘well he’s paying for it, so we’ll take his money. I told GE at the time that this would be a future clinical product. Well, they had a clinical product by May 1999, six years from an idea that was considered ludicrous to the day they actually had a product. I thought that was pretty good.”
3T to 9.4T
The 3T scanner proved to be just a stepping stone: the next step was to get to the highest field scanner for human imaging. During the course of developing 3T, various other projects came online, of which the most influential was Ohio State University’s 8T scanner. They pushed the magnet manufacturers to go to the highest field they were comfortable doing. They also built a 9.4T 30cm magnet for the University of Minnesota.
“Given that they could build a 9.4T 30cm magnet, they were prepared to build an 8T 80cm human system,” says Thulborn. “Unfortunately, at that stage having experienced a 9.4T and an 8T, other influential people decided they wanted to do a 7T, so the manufacturers started building 7T systems. The magnet manufacturers saw that as good for business, but they also saw that they could actually build a 9.4T 80cm magnet.”
Thulborn began to look for an institution that would support a project to build a 9.4T scanner. For human imaging, the smallest bore magnet for a reasonable homogeneity of the magnetic field is 80cm. With an 80cm bore magnet and the current super-conducting wire technology, which is the niobium-titanium wire at 4.2 degrees, a current density will enable 9.4T. “If you try and push the current density higher – you can’t – it won’t be super-conductive,” says Thulborn. “9.4T represents the edge of the envelope for the current super-conducting wire technology.”
UIC agreed to support the 9.4T project through a venture capital project. Thulborn joined UIC in 2000. It took three years to build the magnet and construct the building that would house the magnet; and in September 2004, the 9.4T scanner was born. “It takes a while to assemble this technology,” he says. “You’ve got to build it, and then everything has to go through the FDA. Things don’t go very fast, so we hadn’t done much in four years. But that is only because there is so much paperwork.”